NEWS
A High-Temperature Bulk Superconductor Capable of Generating the World's Strongest Magnetic Field
Masaru TOMITA, Dr. Eng.
Senior Researcher, Maglev Systems Development Department,
(Now visiting at Massachusetts Institute of Technology)
Due to their large flux pinning force, .high-temperature bulk superconductors have a considerable advantage of high critical current densities, even in environments of high temperatures and strong fields. Their ability to generate strong fields has led to expectations of application across a wide variety of areas related to superconductors.
However, a lower level of mechanical strength has been found in such superconductors, resulting in a number of problems including the creation and development of internal cracks due to thermal stress and/or electromagnetic forces, and damage to the superconductor body.
Bulk superconductors have also demonstrated problems at the stage of implementation. For example, during the excitation of a very strong field, the particular superconducting phenomenon of magnetic flux line movement causes heat generation. This leads to heat accumulation within the superconductor due to poor heat release, and superconduction is broken, making field trapping impossible.
We have studied methods of controlling cracks in high-temperature bulk superconductors, and have identified the crack creation mechanism associated with bulk cooling and electromagnetic forces while the field is trapped. Based on this finding, methods of infiltrating cracks with epoxy resin were considered and as a result, higher superconductor strength and durability in the high temperature bulk superconductor were achieved. Additionally, through the technique of impregnating the inside of the bulk body with a metal with a lower melting point, thermal stability has been increased and the improved trapped field exceed the world's highest value of 17 teslas.
This achievement was published in the scientific magazine Nature last year, and attracted global attention as a rational technique to increase strength and durability for the improvement of field generation. It is now incorporated into bulk superconductors for the development of next-generation devices such as the magnets and current lead conductors for Maglev Train, magnetic isolators, NMR (MRI: Magnetic Resonance Imaging), flywheels for electric power storage and superconducting motors. It is also expected to accelerate the development of superconducting application technologies.
In bulk superconductors, electromagnetic forces or thermal stress are active when a field is excited. Insufficient strength in the bulk superconductors can lead to damage and degradation of field characteristics. The technique of impregnating superconductor cracks with epoxy resin in a vacuum has led to a marked improvement in mechanical properties, enabling the superconductors to perform at a practical level.
Observation of the inside of bulk bodies has established that the resin infiltrates through micro cracks and air holes. Such infiltration inhibits the creation and development of cracks due to the increased strength provided by the resin itself as well as the greater bulk body adhesion. Hence, the mechanical properties of the bulk superconductors are improved.
Figure. 1 shows the variation in trapped-field properties and field distribution from repeated trapped-field measurements. Negligible property degradation is seen with resin-impregnated bulk bodies. It is clear that this technique has achieved higher superconductor mechanical strength as well as improvements in the trapped field. Despite this greater mechanical strength, however, breakage was found to prevent trapping at field values greater
than 14T.
Experiments also show that higher field trapping is inhibited when the superconductor internal heat generation associated with magnetic flux movement causes the superconductor to become normal (see Fig. 2). This internal heat generation is due to the lower thermal conductivity of the bulk superconductor compared with metalic superconductor.
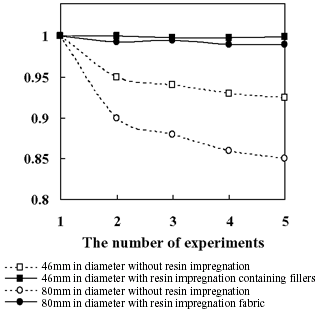 |
|
Fig. 1 | Trapped-field values versus the number of measurements for bulk Y-Ba-Cu-O superconductors and trapped-field distributions. (a) bulk superconductor with resin in the first run, (b) with resin in the fifth run, (c) bulk superconductor without resin in the first run and (d) without resin in the fifth run. |
|
During the excitation of a bulk magnet, an external fluxoid enters the superconductor, and this flux action invariably causes heat generation. The removal of heat through an external cooling medium can relieve the problem,
but continued heat generation results in an increased local temperature. As a result, the superconducting properties in the affected area fall drastically, and breakage is found in superconducting magnets when a large electromagnetic force occurs during local and rapid field changes.
For the purpose of controlling such heat generation, a hole was drilled in the center of the resin-impregnated bulk body and high-thermal-conductivity aluminum was inserted. This was then impregnated with a low-meltingpoint metal (Bi-Pb-Sn-Cd) to achieve higher thermal conductivity and an increased thermal contact area between the metal and the bulk body. Figure. 3 shows the internal structure, a cross-section and an overview, indicating infiltration
of the previously impregnated resin around the bulk body. It also shows the state where the low-melting-point metal has infiltrated the bulk body and the increased thermal contact area between the metal and the bulk body.
This technique of impregnating the bulk superconductor with epoxy resin and low-melting-point metal has resolved both issues found with the mechanical and thermal properties of high-temperature bulk superconductors which were previously seen as major challenges to practical use. This has also enabled the trapping/generation of the world's strongest field of 17.24 teslas at 29K, as shown in Figure 4.
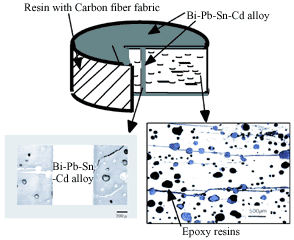
Fig. 3 | Structure and optical micrographs of bulk superconductors with greatly improved in addition to reinforcement for high trapped-field. |
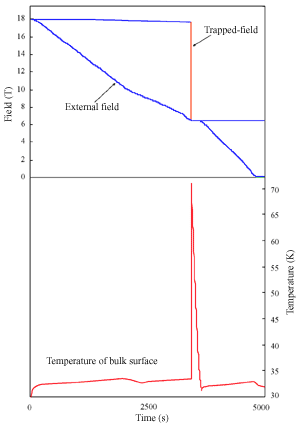 | 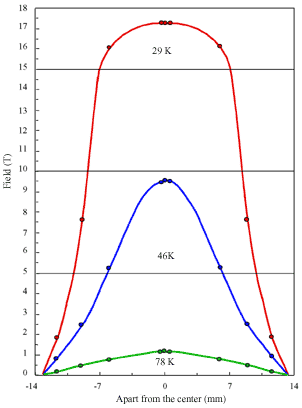 |
Fig. 2 | Variation of the field and temperature in the bulk superconductor with resin impregnation when the external field was reduced from 17.9T to zero. |
|
Fig. 4 | The field distribution trapped by bulk YBCO silver addition samples 26.5mm in diameter with metal impregnation technique at three different temperatures. |
|